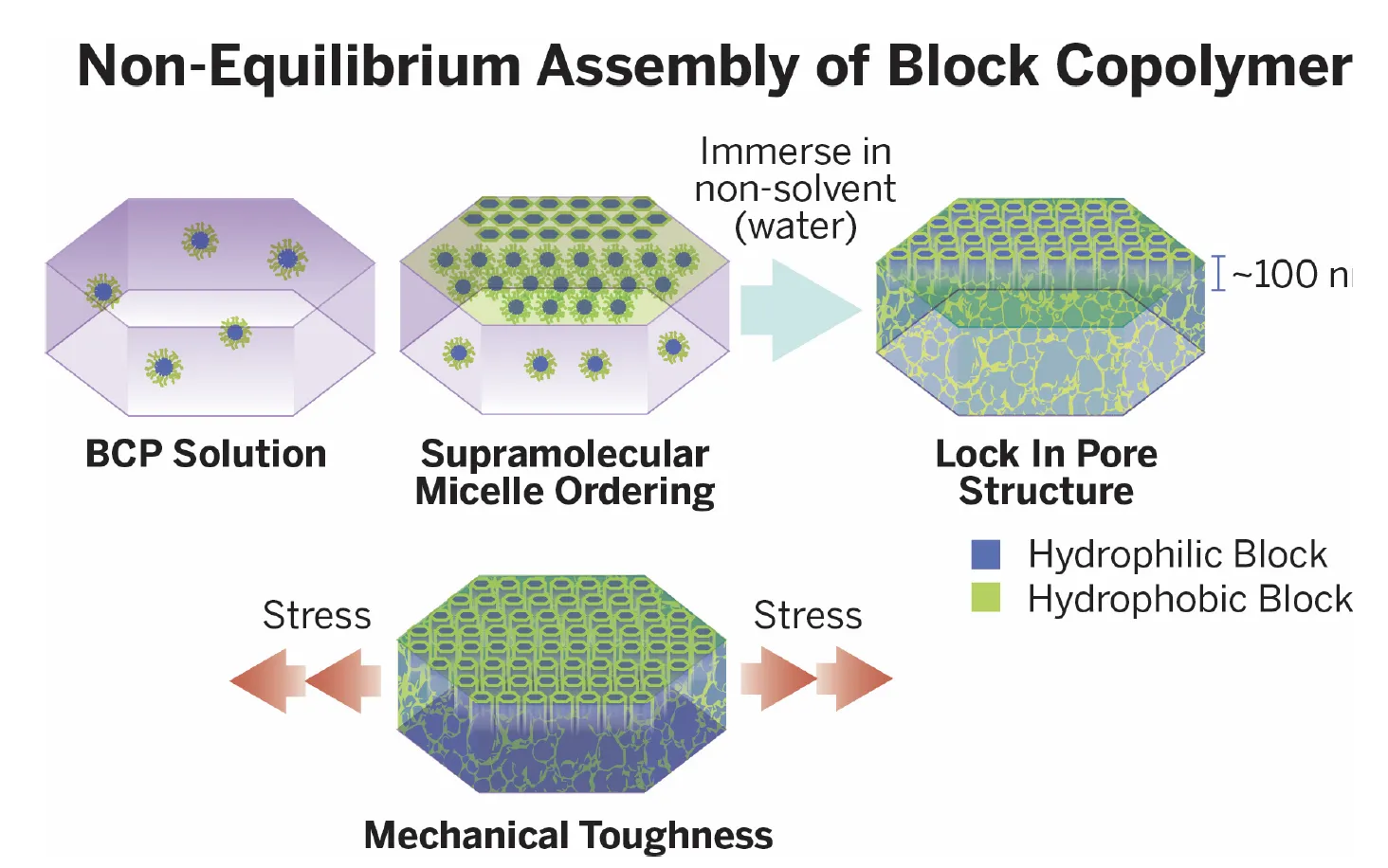
Fig. 4.1. GAP C will use UMCP (Universal Membrane Chemistry Platform) materials to co-designing mechanically robust, permeable, selective membranes for filtering aqueous solutions of MFP (Model Fluid Platform) components. Via systematic experimental and theoretical studies, we will identify the scientific fundamentals underpinning the creation of robust isoporous membranes, gaining rational control over the SNIPS (non-solvent induced phase separation (NIPS) with self-assembly) process to radically transform membrane manufacturing.
Fundamental Science of Robust Membrane Manufacturing
GAP Co-Leaders:
UT Austin: Gabriel Sanoja
UC Santa Barbara: Glenn Fredrickson
GAP Co-Investigators:
UT Austin: Berkin Dortdivanlioglu, Benny Freeman, Venkat Ganesan, Lynn Katz, Nate Lynd
UC Santa Barbara: Todd Squires (UC Santa Barbara),
Lawrence Berkeley Laboratory Advanced Light Source: Greg Su
Problem Statement
As shown in Fig 4.1 (above), GAP C will develop the fundamental knowledge of materials and process science to enable the large-scale manufacture of mechanically durable, high-performance membranes for ultra and nano-filtration of water. Non-solvent induced phase separation (NIPS) is the predominant commercial method to manufacture porous polymer membranes that are thin (i.e., high flux) and selective. In this process, a non-solvent (usually water) is contacted with a film of polymer dissolved in a good (organic) solvent. As the non-solvent diffuses into the film and the good solvent diffuses out, the polymer phase precipitates and ultimately vitrifies, leaving a water-filled pore structure in the wake of the vitrification front. In spite of decades of investigation, NIPS processes are conducted as “black box” operations with little understanding of the farfrom- equilibrium physics connecting process and formulation inputs with membrane microstructure and performance. It is therefore difficult to improve upon the current state of the art and evolve membrane performance to optimal levels of flux and selectivity. NIPS membranes for ultra and nano-filtration typically have one polymer component, usually a polysulfone or poly(vinylidene fluoride) homopolymer, and an asymmetric structure comprised of a gradient in pore sizes across the membrane. The surface layer of the membrane with the smallest pores provides all the selectivity and most of the hydrodynamic resistance to water flow, while the remainder of the membrane primarily serves as mechanical support. Such membranes have the mechanical strength to survive long-duration commercial separations, while offering a reasonable compromise of water permeance against solute size selectivity.
Emerging research on a process that substitutes the homopolymer with a block copolymer, so-called SNIPS (NIPS with self-assembly), has shown considerable promise in creating membranes with dramatically improved flux and selectivity. Well-orchestrated SNIPS membranes have an underlying gradient pore structure like that of NIPS, but a better-organized surface layer (i.e., skin) with a narrow pore size distribution centered in the 10 nm scale range. The high degree of order in the skin arises from microphase separation of the block copolymer. Such “isoporous” membranes break the traditional inverse relationship between flux and selectivity and represent a major opportunity for improvements in membrane performance and the energy efficiency of water purification. To date, SNIPS has been demonstrated on a narrow class of amphiphilic diblock copolymers, generally involving hydrophobic polystyrene (PS) and short hydrophilic poly(4-vinyl pyridine) (P4VP) blocks (i.e., PS-b-P4VP). In SNIPS membranes prepared from casting solutions with solvents favoring the hydrophobic PS block, the short hydrophilic P4VP block lines the pore walls in the skin layer, while the longer PS block forms the inter-pore matrix. Solvents and casting conditions necessary to achieve isoporous membrane structures have been optimized for this specific system, but the resulting membranes are brittle and unsuitable for commercial applications. While the inclusion of rubbery blocks has revolutionized the design of tough thermoplastic elastomers via block copolymer self-assembly, analogous fundamental design rules for toughening porous SNIPS membranes do not exist nor is there a set of design rules that allow efficient navigation of the vast design space to create isoporous, tough membranes.
GAP C will remedy this problem by developing the scientific understanding necessary for SNIPS-based manufacturing of high-performance membranes from universal membrane chemistry platform (UMCP) amphiphilic block copolymers with optimal flux, selectivity, and mechanical properties. GAP C will both develop fundamental understanding of the role of compliant blocks and pores on membrane toughness and crack propagation in order. Further, a new physics-based model of the non-equilibrium SNIPS process will guide the identification of optimal solvents and casting conditions, as well as provide a fundamental understanding regarding the interplay of multi-component diffusion, thermodynamics, self-assembly, polymer entanglement, and vitrification in establishing membrane structure. Altogether, the knowledge gained by GAP C has the potential to enable the large scale manufacture and commercial deployment of polymer membranes with unprecedented separation performance.
The fundamental science questions on isoporous membranes that GAP C will tackle are aligned with the DOE’s Grand Challenges for Basic Energy Sciences, in particular “How do we characterize and control matter away– especially very far away –from equilibrium?” and the Transformative Opportunities for Discovery Science, e.g., “Mastering Hierarchical Architectures and Beyond-Equilibrium Matter.” GAP C is uniquely suited to address the fundamental science of manufacturing, and targets priority research directions of the Transformative Manufacturing BRN, including to “achieve precise, scalable synthesis and processing of atomic-scale building blocks for components and systems,” and “unravel the fundamentals of manufacturing processes through innovations in operando characterization.”
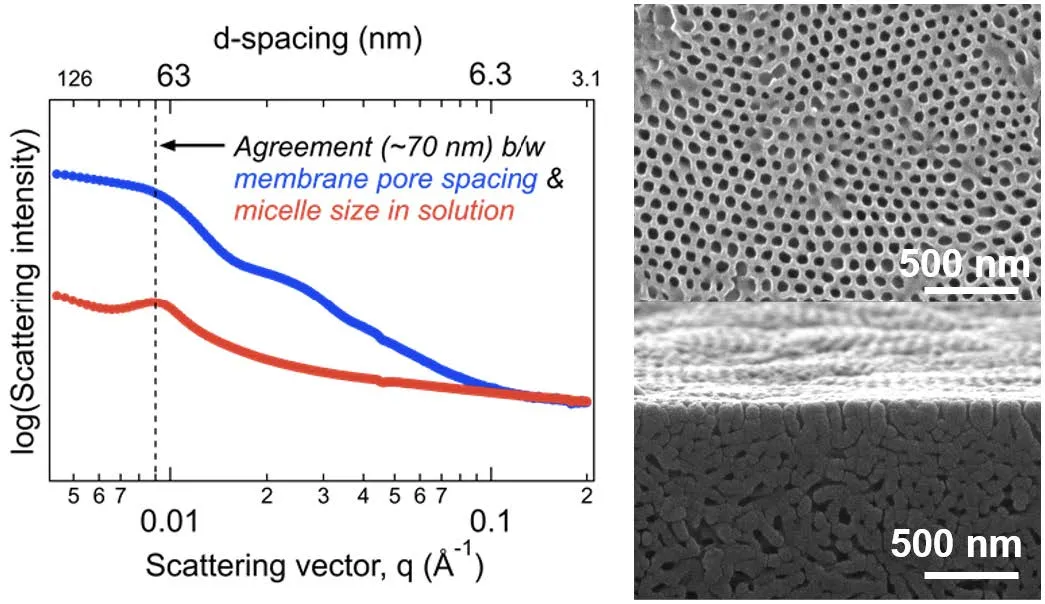
Fig. 4.2. Surface (top) and cross-sectional (bottom) SEM images of an M-WET isoporous membrane prepared via self-assembly + aqueous nonsolvent-induced phase separation (NIPS) from a casting solution of 13 wt. % PSb-P4VP dissolved in a 1:1:1 blend of N,Ndimethyl- formamide, 1,4-dioxane, and tetrahydrofuran. This membrane was prepared with a 20s dry step at 50% relative humidity. Agreement in length scales obtained from SAXS profiles of the micellar casting solution and the final membrane highlight M-WET’s ability to control pore size of SNIPS membranes through directed self-assembly of PS-P4VP micelles in casting solutions.
Research Questions
How do we design and synthesize UMCP polymers that are suitable for SNIPS casting into tough/strong isoporous membranes?
How do we identify scalable, translatable manufacturing processes that can be rapidly adapted to different membrane chemistries?
How do we manage the vast design space of polymer, solvent, and process variables to identify casting conditions that lead to isoporous membranes?
What is the role of pores and hierarchical pore structure on the mechanical properties of membranes?
Research Approach
The GAP C research is divided into three efforts:
Project 1, which integrates modeling and experiment to identify polymers, casting solvents, and conditions that produce isoporous membrane structures;
Project 2, which applies advanced characterization techniques to monitor the in situ formation of hierarchical membrane structure during SNIPS and in operando structure and transport characteristics of the finished membrane; and
Project 3, which seeks to understand how the mechanical properties of membranes are connected to hierarchical pore structure, and to variations in polymer chemistry, architecture, and process conditions.
GAP C, Project #1: Design and Preparation of Isoporous Separation Membranes
GAP C research will leverage our early experience in performing the SNIPS process with PS-b-P4VP and related systems (cf. Fig. 4.2) towards the reproducible fabrication of isoporous asymmetric membranes from UMCP materials. Experimental efforts will be directed at understanding and harnessing the nonequilibrium phenomena that will enable the manufacture of isoporous membranes with tunable pore size and size distribution. New UMCP copolymers will be characterized for solution phase ordering via X-ray scattering to narrow the casting solution design space to solvents best suited for inducing self-assembly (Su). UMCP variants capable of forming isoporous asymmetric membranes will undergo further characterization of thermomechanical properties via tensile testing, dynamic mechanical analysis, and thermogravimetric analysis of dense films (Sanoja) to inform synthetic efforts aimed at improving material strength/toughness and thermal stability (Lynd). Isoporous UMCP membrane structures will be identified and characterized via electron microscopy, and X-ray scattering. Their separation performance, namely pure water permeance, molecular weight cutoff, solute rejection, and fouling behavior, will also be measured in the IF (Freeman).
The experimental program on SNIPS will leverage a parallel theoretical and computational effort to develop and exploit models of the dry evaporation and wet immersion steps of SNIPS. Phase field models will be constructed (Fredrickson, Ganesan) based on multi-component fluctuating diffusion equations (Model B dynamics) for the densities of solvent, non-solvent, co-solvent, and individual block species. The Rouse model is used to compute the Onsager mobility tensor in the dynamical equations. Structural arrest is described by the sigmoidal increase in local friction experienced by solvents and polymer segments when a glass transition threshold is crossed. Such a model produced realistic asymmetric membrane structures in prior NIPS simulations. Unlike that work, where the chemical potential gradients driving multicomponent diffusion were derived from a phenomenological Flory-Huggins-de Gennes free energy functional, the thermodynamic forces will be numerically computed using self-consistent field theory (SCFT). This approach embeds a rich thermodynamic basis for the model that has a molecular origin faithful to the multiblock polymer architectures deployed. The resulting dynamic SCFT framework (DSCFT) can capture both the macrophase and microphase separation processes involved in SNIPS.
Modeling of the dry and wet steps of SNIPS will occur sequentially. The dry evaporation protocol will follow previous work on modeling solvent evaporation from thin block copolymer films. Key variables to explore are the evaporation time, the solvent volatility, and the block selectivities towards solvent, cosolvent, and air. The morphological output from the dry evaporation step provides the initial condition for wet immersion, wherein the air phase is replaced by a non-solvent bath that is permitted to diffuse into the film and initiate macrophase separation. A preliminary example of the output from such a two-step simulation is shown in Fig. 4.3 for a symmetric (i.e., 𝑓A = 0.5) AB diblock copolymer in a film with a nonselective solvent S (equal Flory interaction parameters, 𝜒AS. = 𝜒BS.). In this simple two-dimensional example, the non-solvent is also non-selective for the A and B blocks (i.e., 𝜒AN = 𝜒BN). Fig. 4.3 reveals a skin layer with well-organized vertical diblock lamellae transitioning below into a membrane structure with solvent-filled macropores. Future investigations will extend to three dimensions, focus on the UMCP architecture, and include vitrification when the concentration of the matrix-forming block locally exceeds a glass transition threshold.
Since GAPS A and B will incorporate functional groups to impart selectivity to UMCP membranes via chemistry that can be implemented in either the porous or non-porous formats, the SNIPS/NIPS manufacturing process must be robust to these additional groups. Yet, we have found that the inclusion of a relatively small pendant group has a profound effect on the Flory interaction parameter87, potentially altering the thermodynamics of phase separation/membrane formation substantially. An important component of the modeling effort will be to establish a reliable method of estimating the Flory interaction parameters that are inputs to the dynamical theory and describe the pairwise interactions among polymer segments, solvents, co-solvents, and non-solvent. Because the chemical variations that will be explored in the project are wide-ranging, an in silico method that requires no experimental input will be pursued. Specifically, structure factors obtained from all-atom molecular dynamics (MD) simulations of short polymer blocks mixed with solvents or low molecular weight polymers will be fit to analytical random phase approximation (RPA) structure factors to extract 𝜒 parameters (Ganesan, Fredrickson). Although this procedure has precedent in the literature, we have recently discovered that substituting intramolecular scattering information obtained from the MD simulations for theoretical Debye functions yields much more accurate values of 𝜒.
The experimental and theoretical components of Project 1 will be closely coordinated, with theory informing experiment of the most promising polymer designs and choices of solvents, casting conditions, and with experiments providing a platform for validating the assumptions and parameters of the underlying models.
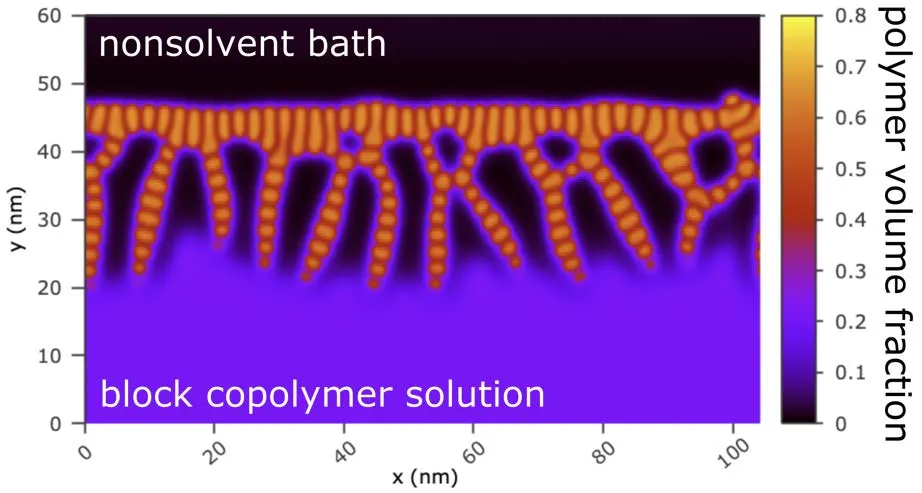
Fig. 4.3. Morphology obtained from dynamic SCFT simulation of mass transfer at an AB block copolymer solution/nonsolvent bath interface, for 𝑁 = 50, 𝑓A| = 0.5, 𝜒AB = 1, 𝜒AS. = 𝜒BS = 0.8, and 𝜒AN = 𝜒BN = 1.048. The initial volume fractions in the bulk of the polymer solution film are 𝜙P = 0.2 (polymer), 𝜙S = 0.7 (solvent), and 𝜙N = 0.1 (nonsolvent).
Project 2: Operando Characterization of Assembly Pathways in Integral Asymmetric Membranes
Project 2 of GAP C will experimentally unveil the formation process of integral asymmetric UMCP-based membranes. Project 1 (Fredrickson, Ganesan) will provide preliminary estimates of the kinetic evolution of self-assembly and phase separation, two inherently non-equilibrium processes that are challenging to predict and control/reproduce. Su, Katz, and Crumlin will develop operando scattering tools within the IF that will be used in GAP C to track each stage of the SNIPS process on the sub-micron scale, addressing knowledge gaps that connect block copolymer chemistry, architecture, and processing to isoporous membrane structure. To complement this sub-micron characterization, Squires will develop microfluidic interferometric techniques that track the spatio-temporal evolution of the evaporating solvent and infiltrating non-solvent under controlled conditions across the thickness of the film, directly visualizing both wet and dry steps.
The main steps of the SNIPS process are block copolymer micelle formation in solution, micelle assembly into a microphase-separated skin during solvent evaporation, and nonsolvent-induced macrophase inversion (i.e., NIPS). In situ characterization tools will be employed for each of these three steps.
Step 1: Micelle formation - In situ X-ray scattering techniques (developed in the IF and utilized here) will reveal structure formation and kinetics. Block copolymer micelle structure and size in solution dictate the characteristic pore size and spacing within the skin layer (Fig. 4.2). X-ray scattering of block copolymer micelles in solution (see discussion in the IF Section 5.1.4 and Fig. 5.6) will track the size of block copolymer micelles and inform optimal solvent compositions. Moreover, in situ liquid resonant soft X-ray scattering (RSoXS, developed in the IF), will be used to differentiate the spatial extent of the core vs. shell regions of micelles (Su, Katz). These findings will guide Project 1 experimental efforts to design UMCP SNIPS casting solutions for desired membrane pore sizes and chemistries.
Step 2: Solvent evaporation - After membrane casting, micelles merge as the solvent evaporates, which templates the morphology of the isoporous skin layer in a non-equilibrium state. Understanding the fundamentals of this process requires operando measurements that reveal the evolving concentration profiles of solvent and the structural evolution of the skin layer. This will connect features of solution morphology, e.g., the size of the micelle core, to the morphology of the isoporous skin layer. Such measurements provide feedback to guide block copolymer synthesis (Lynd), validation for physics-based computation (Fredrickson, Ganesan), and feedforward to full membrane structure, mechanical properties, and separation performance (Freeman, Sanoja). The operando X-ray scattering device will be developed in the IF with appropriate time and length scales to be leveraged for tracking the morphology evolution during the solvent evaporation step (cf. IF Section 5.1.4 and Fig. 5.6).
From our simulations, skin layer ordering and growth are driven by concentration profiles that change both spatially and temporally. These rates will be measured using a microfluidic interferometry technique (Squires), (cf. Fig. 4.4), to track the solvent profile across precursor films exposed to a flowing gas of controlled composition (e.g., specified vapor pressure of organic solvent or H2O), with ~1 μm spatial and 100 ms temporal resolution.
Step 3: Macrophase inversion - In situ characterization will also include the observation of structure evolution during nonsolvent immersion. Immersion in a nonsolvent produces the open, porous structure below the skin layer due to macrophase separation between the nonsolvent and polymer. In situ X-ray scattering (developed in the IF by Katz and Su) will reveal if changes in the self-assembled isoporous layer occur upon nonsolvent immersion. Operando characterization of asymmetric membrane formation will be complemented by ex-situ interrogation of previously formed membranes to connect processing conditions to the resulting membrane structure/performance. The UMCP platform provides a route towards isoporous membranes with functionalizable hydrophilic pore walls. Synchrotron infrared nanospectroscopy (SINS) and resonant X-ray scattering (developed in the IF) will be used to map nanoscale morphology with chemical sensitivity, through unique IR signatures of specific functional groups and tunable scattering contrast, respectively, providing unprecedented simultaneous resolution of morphology and chemical structure.
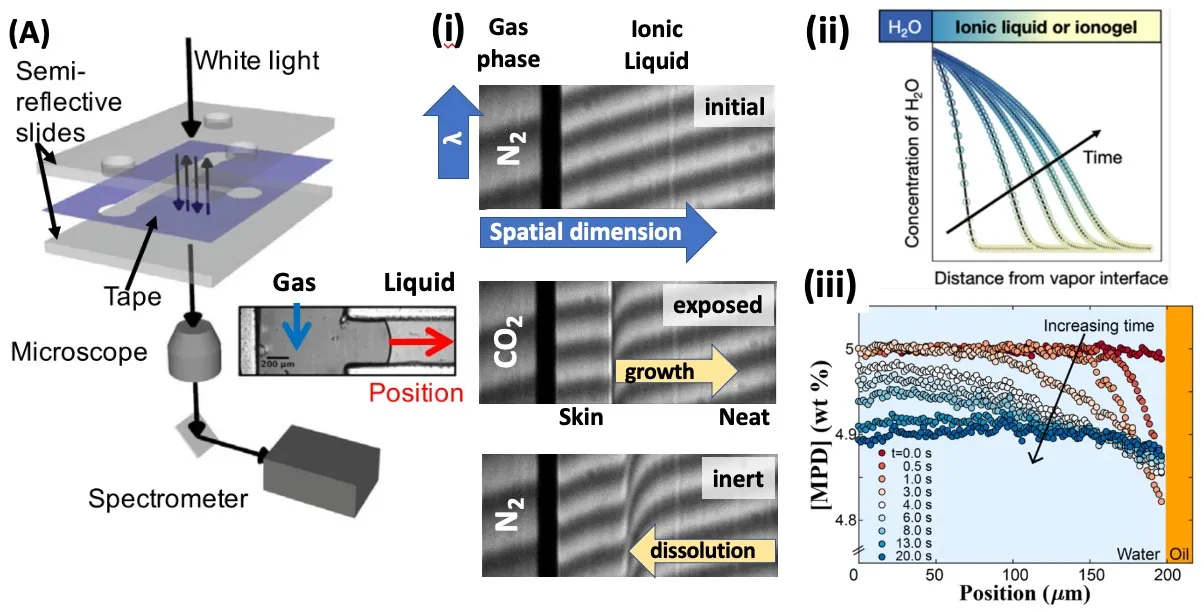
Fig. 4.4. Microfluidic interferometry tracks spatio-temporal evolution of concentration during film drying/ “skin” layer growth.
Project 3: Mechanics of Isoporous Membranes
Development of next generation isoporous membranes requires co-design for optimized selectivity, permeability, and mechanical properties. While much is known about the design of mechanically tough polymers, we need to understand the specific role of pores in the fracture process to design tough membranes. With this understanding, implementation of known block copolymer anchoring and other nanometer scale geometrical features to improve fracture energy, yield stress, and fracture strain is possible.
To understand the role of pore structure on the mechanical properties of membranes, a UMCP series of varying porosity will be produced by SNIPS, equilibrated in water, and inflated until failure with a membrane inflation apparatus shown in Fig. 4.5A and 4.5C (Sanoja). The resulting apex stress-strain curves will be used to validate and inform our simulations and determine mechanical properties including the biaxial modulus and work of extension until failure (Dortdivanlioglu). By identifying the critical pore size distribution at which the work of extension until failure of an isoporous membrane matches or outperforms that of a dense membrane, membranes will be prepared with improved transport properties and excellent mechanical integrity. A recent review by Long et al. argued that dense elasto-plastic materials like block copolymers with cracks of order 1 mm behave as pristine, suggesting that pore sizes from 10 nm to 1 mm should not affect the mechanical properties279. However, this prediction assumes that mechanical properties like the fracture energy, yield stress, and fracture strain are insensitive to specimen geometry and hierarchical structure. Experimental validation is required in thin, porous membranes where confinement effects and stress inhomogeneities could be important.
UMCP membranes will also be cast on deformable substrates (e.g., copper grids), equilibrated in water, and equibiaxially deformed until failure (Fig. 4.5B, D) (Sanoja, Fredrickson). By monitoring the crazing and failure strains using in situ optical and scanning probe microscopy, and SAXS during tensile testing, fracture mechanisms of membranes will be unveiled, as will be molecular designs that delay craze nucleation and growth under equibiaxial loads.
Understanding the brittle-to-ductile transition of membranes is important to prevent catastrophic failure, but membrane lifetime is often controlled by creep under fixed sub critical loads. To evaluate this transition, UMCP membranes will be inflated to a fixed apex stress, with the apex strain monitored as a function of time (Sanoja). In collaboration with the IF, we will also evolve the UMCP to include rubbery blocks within the polystyrene membrane matrix to impart compliance. Other forms of physical and chemical cross-links within the matrix will also be explored as a part of the UMCP evolution to a more robust material. Multi-scale finite element (FEM) models will complement experimental efforts to understand the role of microscopic pore structure on the macroscopic mechanical properties of UMCP membranes (Dortdivanlioglu). At the mesoscopic scale, Representative Volume Elements (RVE) of the hierarchical structure will be generated to model the pore-polymer interfaces connecting the walls of the pores with the bulk of the membrane. The bulk and interfacial properties will be informed by atomistic simulations (Dortdivanlioglu, Ganesan), and the RVEs used to construct a myriad of constitutive stress-stretch relations that describe the gradient in mechanical properties from the top to the bottom of the membrane. Integrating such mesoscopic information into macroscopic FEM models via numerical FE2 homogenization methods284 will provide a multi-scale picture of the role played by pore structure and interfacial segregation of block copolymers in the mechanical properties of UMCP membranes. Notably, this multi-scale FEM model can be used to describe anisotropic, capillary, or inelastic behaviors at the pore-polymer interface, meaning that it can both enable novel phase-field models for predicting the onset of crack propagation and aid in designing selective and permeable membranes that resist equibiaxial loads under wet operating conditions.
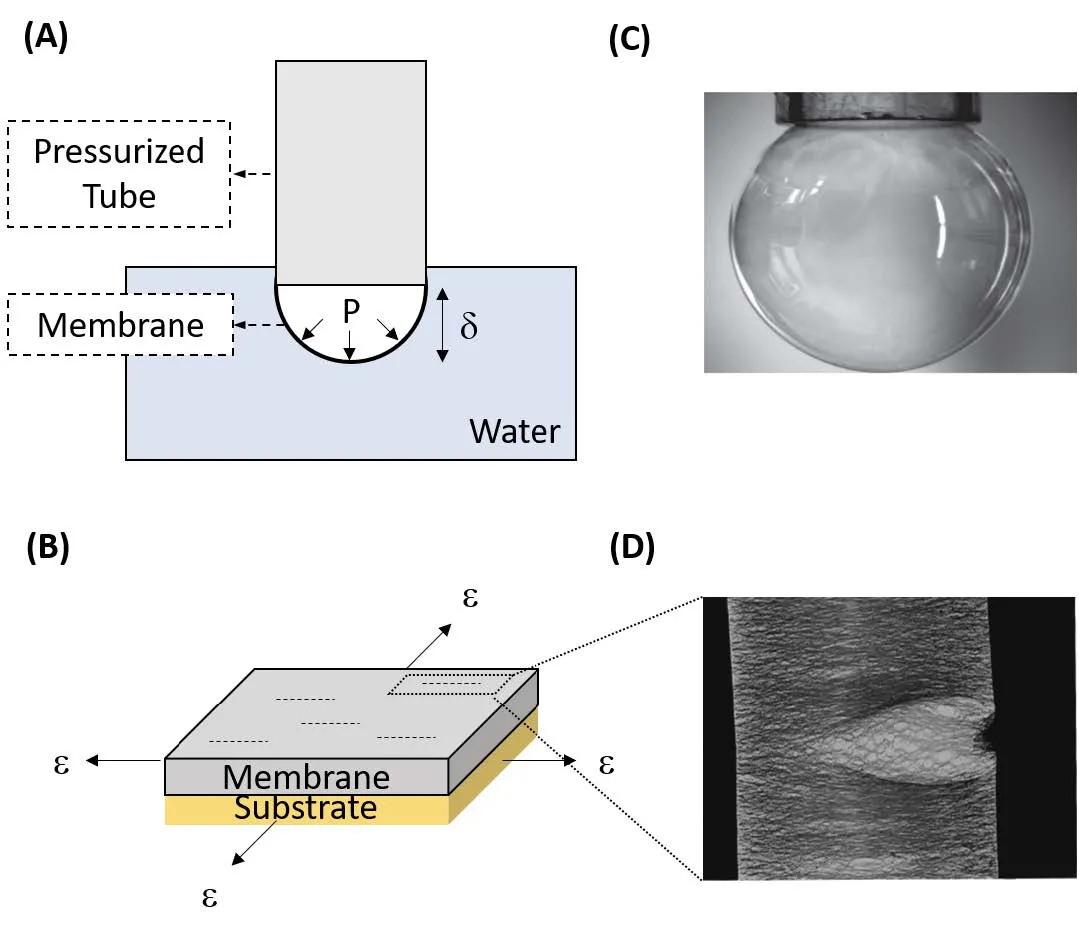
Fig. 4.5. Mechanical properties will be evaluated by (A) inflating (B) equibiaxially deforming membranes while monitoring (C) inflation deflection, and (D) crack nucleation and growth.
Research Highlights - GAP 3 (M-WET project years 2018 to 2021)
Compositional Control of Crosslinked Polyether Networks
Cross-linked polyether networks of varying hydrophilicity have been synthesized using photo-induced cationic polymerizations and will be used to determine how polyether composition can influence water dynamics.
Salt permeation in ligand-functionalized polymer membranes
We show that partitioning dominates over diffusivity to dictate ideal salt permeation in ligand-functionalized polymer membranes.
Connecting Solute Diffusion to Membrane Morphology
Computer simulations reveal that solute diffusion strongly correlates with simple geometric measures of porous triblock copolymer membranes.
Influence of Membrane Architecture on Fluid Transport
Self-Consistent Field and Dissipative Fluid Dynamics simulations and polymer synthesis/membrane characterization probe the impact of pore morphology and pore wall coatings on water transport in a nanoporous membrane.
Role of Side-Chain Architecture in Poly(ethylene oxide)-based Copolymers
The design, synthesis, and characterization of a series of copolymers comprising a poly(ethylene oxide) backbone with discrete linear/cyclic oligo(ethylene oxide) side chains is reported.
Simulations Reveal Asymmetric Membrane Formation Mechanisms in Nonsolvent-Induced Phase Separation
Phase-field simulations demonstrate that mass-transfer-driven spinodal decomposition, thermal fluctuations, and structural arrest are essential to the formation of asymmetric polymer membranes in the Nonsolvent-Induced Phase Separation process.
Versatile Synthetic Platform for Building Membrane Libraries
A robust and efficient synthetic strategy permits development of wide libraries of membrane structures with well-controlled functionality.