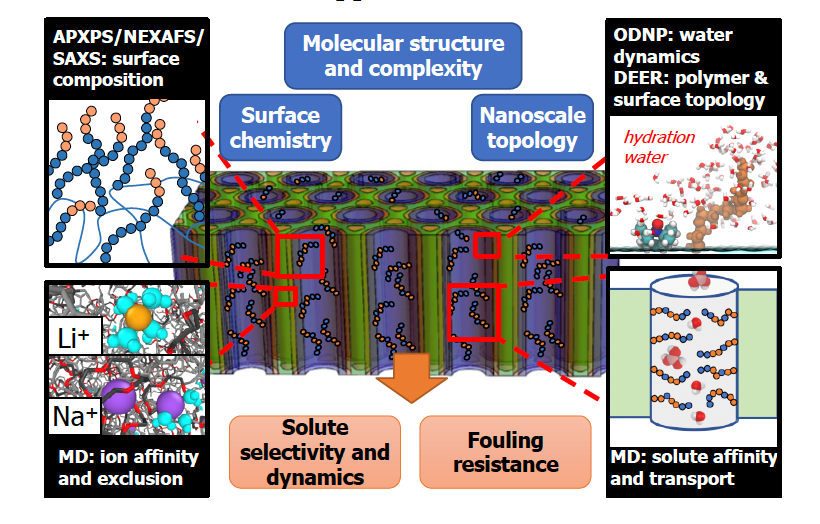
GAP A will integrate state of the art spectroscopic tools to measure surface chemical compositions, including solutes, as well as ODNP to probe water dynamics and double electron–electron resonance (DEER) spectroscopy to quantify surface polymer conformational landscapes. Complementary MD simulations will compute ion and solute affinities/activities at surfaces, as well as water structural, thermodynamic, and dynamic properties.
Structure and Dynamics of Water and Solutes Near Interfaces
GAP A Co-Leaders: Lynn Katz (UT) and Scott Shell (UCSB)
GAP A Co-Investigators: Ethan Crumlin (LBNL), Graeme Henkelman (UT), Nate Lynd (UT), Rachel Segalman (UCSB), Greg Su (LBNL)
Problem Statement:
Our understanding of membrane processes and phenomena, including transport, partitioning, selectivity, and fouling, crucially depends on knowledge of interactions of water and solutes with membrane interfaces to permit design of functional surfaces with targeted solute partitioning, fouling resistance, and selective solute transport. Conventional approaches to membrane design relying on heuristics to adjust surface hydrophobicity, charge, and roughness have faced barriers in simultaneously engineering desired antifouling, transport, and permeability-selectivity properties. Even advances using heterogeneous surfaces rely on heuristic interface design. For example, recent efforts suggest that amphiphilic surfaces with hydrophobic and hydrophilic regions are key to achieving both fouling resistance and release, while other studies suggest that highly hydrophilic zwitterionic surfaces confer strong fouling resistance through the formation of a tightly bound and networked water hydration layer. However, in either design, the molecular scale chemical and structural properties responsible for fouling resistance are not clear, and hence the optimization and scaleup approach remains heuristic. To effectively inform membrane surface design, we must understand what surface properties define hydrophobicity at the nm-scale. How do hydrogen bonding, electrostatic, and other interactions determine surface hydrophilicity, and do different hydrophilic chemistries impact the hydration layer and fouling in qualitatively distinct ways? How does the specific chemistry of e.g., a charged surface group tune hydration water and solute affinity? How does surface softness and topology impact solute affinity? Without a foundational understanding of the molecular mechanisms underpinning water, solute interactions/binding, and surface solvation thermodynamics, current membrane design remains tied to Edisonian trial and error approaches.
Objective:
The objective of GAP A is to develop this critical missing fundamental picture of surface-water and surface-solute watermediated interactions that identifies molecular mechanisms underlying solute affinity. We focus on heterogeneous interfaces that remain poorly understood compared to the large body of work that has characterized highly idealized, homogeneous interfaces. Quantitative studies of heterogeneous surface systems will generate design rules for macroscopic and molecular-scale surfaces to (1) engineer surfaces with targeted hydration properties, (2) control solute-surface affinity, and (3) manipulate water and solute affinity and repulsion to identify conditions to optimize solute/ion adsorption (non-fouling, separation) and desorption (foulant release, membrane cleaning). Together, these learnings will inform computational inverse design of membranes with tailored properties. Such knowledge gain also has wide-ranging influence on many other challenges, including e.g., the design of active functional surfaces (e.g., antifouling, antimicrobial, biocatalysis), novel adsorbents, electrochemical energy storage, and biomolecular separations and sensors.
Research Questions
We focus on fundamental knowledge gaps in understanding the response of water and solutes to surfaces at the nanometer scale, and the effect of these thermodynamic, dynamic, and structural responses on macroscopic properties of transport and solute affinity/adhesion. We address the roles of chemistry (e.g., hydrophobicity, charge), topology (e.g., polymer brush density), heterogeneity (e.g., mixed composition), and solute identity (chemistry, size, charge). Our questions focus on three levels of understanding:
- How does membrane surface chemistry and topology impact the surface hydration water layer and its properties? What is the effect of surface chemistry, flexibility and topology, chemical group composition and spatial presentation on hydration layer water structure, thermodynamics, and dynamics? What defines a “bound” water layer in terms of these properties?
- How does surface chemistry and topology tune the affinity, activity, and diffusivity of solutes at the interface? How do specific surface chemistries and compositions impact solute affinity? What structural or dynamical properties of the water hydration layer signal solute affinity and are important to control? How are these behaviors modulated by solute type (e.g., size, hydrophobicity)?
- How does surface chemistry and topology impact ion hydration, pairing, activity, and dynamics at the membrane surface? Ions are complex solutes due to their strongly bound hydration shell, ion pairing, and long-range electrostatic interactions. How does ion hydration and pairing change near the membrane surface? How does polymer hydrophobicity, charge density, and hydration impact ion affinity and dynamics? How do complex salt mixtures and high salt concentrations alter surface-ion interactions?
Research Approach
Our unique suite of computational, synthetic, and characterization tools75 allow us to gain critical molecular insight to surface and interfacial interactions that control solute partitioning, transport, and fouling. GAP A will use the non-porous UMCP platform to rapidly screen the role of functional groups such as amphiphiles, hydrogen bond donors, charges, and zwitterions on water hydration and dynamics and solute interactions (Segalman, Hawker). This platform is further capable of seamlessly integrating experimental probes, and featuring the display of functional groups on various surfaces, micelles or particles in solution. Atomic and molecular scale characterization via simulation (Shell, Henkelman), advanced NMR and EPR techniques (Han), and spectroscopic analysis (Katz, Crumlin) of the thermodynamic and dynamic interactions of these moieties with MFP solutes will integrate with selectivity design in GAP B and macroscopic fouling characterization (Katz, Su) in the IF.
Project #1: Surface Design to Control Hydration Water Structure, Thermodynamics, and Dynamics
What is the precise nature of the hydration layer or “barrier” that has been assumed to impact membrane properties? How can this hydration layer be tuned via surface chemistry? We will use the unique toolset established in our earlier M-WET work to probe interfacial water properties on the molecular scale necessary to understand and ultimately design surfaces to program surface water. It is critical to explore and understand the breadth of functionalities necessary to create programmable surface interactions, to ultimately design membranes that are both selective and robust. Using our materials platform capable of producing multifunctionality (Segalman/Hawker), we will experimentally and computationally quantify the role of chemical and topological surface heterogeneity on hydration water dynamics, structure, and thermodynamics. In particular, we will test the idea that a strongly hydrogen bonded hydration water network (i.e., a highly thermodynamically stable hydration layer) is key to antifouling, by comparing hydrophilic surfaces bearing hydrogen bond donors to hydrophilic surfaces without. Similarly, we will probe the role of charges and zwitterions on hydration and water dynamics. These functionalities will first be displayed on water soluble polymers bearing spin probes to understand their influence on local water dynamics. Next, these functionalities will be displayed on micelles where the hydrophobic core forces the display of the surface groups on molecularly flat surfaces at the nanometer scale. Finally, the same functionalities will be displayed in a hydrogel, formatted as a smooth membrane-like surface and as 100 micron particles suspended in water within a capillary suitable for NMR and X-ray characterization.
With these model surfaces, we will leverage the unique suite of tools established in M-WET to address the critical question of how surface chemistry and topology impact measured structural, thermodynamic, and dynamic properties of the membrane hydration layer. Overhauser Dynamic Nuclear Polarization (ODNP) (developed by Han) will probe hydration dynamics near surfaces (appropriately spin-labelled) in both suspended functionalized hydrogel particles and micelles. Specifically, we anticipate that functional groups including fixed charges and hydrogen bond donors will alter the dynamics of the surrounding water. Measured ODNP water diffusivities will then be directly compared to Shell’s molecular dynamics (MD) simulations that quantify water diffusivities as well as their relationships to water structure (e.g., tetrahedrality, H-bonding) and thermodynamics (e.g., solvation energies/entropies, activity). Electronic structure and quantum chemical calculations (Henkelman) will capture the role of ions and charged surface interactions with water and will assist in the parameterization of MD force fields. Measured water dynamics will be complemented by depth-dependent chemical composition and water content in analogous thin films via Ambient Pressure X-ray Spectroscopy (APXPS) (Crumlin). Furthermore, Su will use surface-sensitive near-edge X-ray absorption fine structure (NEXAFS) spectroscopy to decipher functional groups and polymer blocks to quantify surface composition in the top few nanometers of a polymer film. GAP A will leverage powerful characterization methods developed within the IF to further probe surface morphology including nanoscale imaging and scattering methods. By systematically linking surface composition to hydration water response, we will discover design rules for programming hydration water properties and hence modulating solute affinity.
Project #2: Molecular Determinants of Solute Affinity at Heterogeneous Interfaces
Our second focus is understanding how surface chemistry and heterogeneity impact the thermodynamic affinity of solutes to membrane interfaces. We will use the same model surfaces proposed in Project 1 to experimentally and computationally characterize the affinity of MFP solutes to them, establishing a rational framework for engineering solute affinity and repulsion at membrane interfaces. We hypothesize that the hydration water response to a surface (e.g., hydration water structure, dynamics) offers key signatures of solute affinity. Thus, we will couple the hydration water insights from Project 1 to solute affinities for a range of MFP solutes, focusing in particular on several important solute families: (1) aromatics (benzene, toluene, phenol, benzonitrile, xylenes, naphthalene, dioxane) that represent contaminant chemistries of concern and that allow us to systematically modulate hydrophobic interactions, (2) linear aliphatics and alcohols that appear in oil foulants and can probe chain length variations, (3) short polypeptides as protein mimics with varied hydrophilic phobic composition, (4) borates and arsenous acid that form molecularly small, neutral species that are difficult to separate, and (5) silicates that aggregate proteins and NOM on membrane surfaces with inter-solute interactions that apparently increase fouling beyond the sum of the two foulants.
We will characterize interfacial solute adsorption as a function of surface chemistries in functionalized hydrogels using a unique workflow: APXPS measurements (Crumlin) will measure precise, depth-dependent concentrations of solutes at surfaces with varying levels of hydration. Su will use NEXAFS spectroscopy at different detection modes with varying surface sensitivity to unveil solute and polymer composition as a function of depth, while Katz will characterize solute affinity (adsorption) in the bulk membrane interior for a variety of background water qualities ranging from simple single solute systems of varying ionic strength to multi-solute systems. This work will be complemented by resonant soft x-ray reflectivity, a unique capability at ALS, which leverages the same energy-resolved chemical sensitivity of NEXAFS spectroscopy and can quantify the depth-resolved composition of specific solutes in a polymer film with nm resolution. Simultaneously, ODNP measurements by Han, both at the surface of an analogous hydrogel particle and in the interior, will report on changes in hydration shell dynamics in response to solute adsorption. Simulations by Shell will simultaneously compute the spatially-dependent solute excess chemical potential and activity coefficient, from bulk water, to the membrane interface, and then in the membrane interior. The key observables to compare are the surface partition coefficient K and concentration profile c(z). Shell’s computational inverse design methods combining simulations, machine learning, and optimization algorithms will be used to identify membrane surface chemical compositions or peptoid sequences that minimize surface affinity of representative small foulants.
Project #3: Ion Hydration, Thermodynamics, and Dynamics in Networks and at Interfaces
Our final project in GAP A focuses on improved understanding of the impact of aqueous ion interactions (ion hydration, ion pairing) and functionalized membrane material properties (water density/hydrophobicity, charge density) on ion affinity, dynamics, and membrane electrostatics (Donnan Potential, dielectric constant). The importance of hydration and ion-pairing on membrane selectivity for ionic solutes was a theme that emerged in a number of M-WET projects including Li+/ Na+ selectivity in crown ether-functionalized membranes and Na+/Mg2+ in Nafion, and NaCl/Na2SO4 in Nafion. Here, we propose to understand the impact of surface chemistry on ion hydration and ion pairing. In-situ APXPS can quantify the Donnan potential and ion transport as a function of ionic strength, and hence enables us to refine predictive models of ion selectivity for varying functional groups. X-ray absorption fine structure spectroscopy (NEXAFS/EXAFS) will help reveal local environments around specific ions and nearest neighbor coordination shells.
We will incorporate functional groups such as sulfonate, carbonate, imidazoles, phosphonates, amines (secondary and tertiary) and zwitterions into carefully controlled hydrogels to evaluate their relative affinity for a range of cations and anions including alkali metals (Na+, Li+, Cs+), alkaline earth metal ions (Mg2+, Ca2+, Sr2+), halides (F-, Cl-, I-), and oxyanions (nitrate, phosphate, arsenate, sulfate, borate). Both concentration and ionic strength will be varied to assess the impact of ionic concentration on hydration and solute affinity. Macroscopic studies of material properties will be fully characterized in conjunction with the IF using crossflow rejection experiments, concentration gradient-driven permeation experiments, and macroscopic water uptake. Equilibrium sorption experiments will be conducted to determine the solute affinity of each ion/functional group. These data will provide the basis for comparison to molecular dynamics simulation to inform our understanding of the relationships between ion hydration, ion pairing, and changes in water dynamics associated with surface functionalization and charge density.
In-situ characterization of these materials using APXPS will allow us to understand the surface chemistry, solute activity within the membrane, and also the ion solvation environment, revealing an atomistic understanding for macroscopic phenomena. We have developed a new flow cell geometry that allows us to change the liquid on the backside of the membrane to enable the direct observation for ion, solute, solvent transport as a function of time through the membrane. The true power of this capability is that we can observe changes in the Donnan potential and surface chemistry transiently, allowing us to explore both thermodynamic and kinetic properties through the same experimental configuration.
We aim to further understand how the electrical potential distributions at the membrane/solution interface is influenced by size, charge, and chemical interaction of ions. For example, our preliminary work revealed the different ion-membrane interaction induced between Na+ and Cs+ chloride salts. Both membranes equilibrated in the respective salts demonstrate predicted Donnan potentials within the membrane, where the Cs+ ion follows the similar trend and the Na+ ion does not. This behavior reveals unique insight regarding ion-membrane interactions, (de)solvation, and its impact (or lack thereof) on the electrical potentials developed. We propose to further apply this approach to complex ions such as sulfates, phosphates, and nitrates, all of which change the ion size, shape, charge density, and solvation environment which can influence ion pair formation, water content and the degree of interaction within the membrane. These properties can play a critical role in influencing the Donnan potential in ways yet to be directly explored. This will be complemented by ODNP measurements (Han) on micelles bearing fixed charges (as described in Project 1) to understand the role of solute ions such as alkali metals, alkaline earth metals, and halides as they compete with hydration water to interact with the membrane surface. Mixtures of mono and divalent salts (1:1, 1:2 and 2:1 ratio of anion:cation) with varying propensity for ion pairing will also be studied. MD simulations by Shell will provide a powerful tool for understanding ion surface binding, mechanisms of diffusion, diffusivities, ion-pairing, and importantly, how these properties vary with both the anion and cation species and the structure of the membrane. The Henkelman group will use density functional theory (DFT) to simulate detailed ionic interactions beyond MD, including variable charge states and polarizability. Machine learning (ML) corrections to MD force fields, based on DFT calculations will enable high-accuracy modeling using neural-network potentials.
GAP Attack Platform A
-
Ethan Crumlin
GAP A Co-Investigator, IF Co-Investigator
-
Graeme Henkelman
GAP A Co-Investigator
-
Lynn Katz
Associate Director, GAP A Co-Leader, GAP B Co-Investigator, GAP C Co-Investigator, IF Co-Investigator
-
Nate Lynd
GAP A Co-Investigator, GAP C Co-Investigator, IF Co-Leader
-
Rachel Segalman
Associate Director, GAP A Co-Investigator, GAP B Co-Leader, IF Co-Investigator
-
Scott Shell
GAP A Co-Leader
-
Greg Su
GAP A Co-Investigator, GAP C Co-Investigator, IF Co-Investigator
Research Highlights - GAP 1
The Donnan Potential Revealed
Using tender-APXPS, we have directly measured the Donnan potential at the membrane/solution interface for CR-61 membranes equilibrated with NaCl and MgCl2 solutions.
Effects of Amphiphilic Polypeptoid Side Chains on Polymer Surface Chemistry and Hydrophilicity
In situ ambient pressure X-ray photoelectron spectroscopy (APXPS) reveals that polypeptoid-modifications can alter polymer interactions with water and instigate surface restructuring.
Sequence modulates polypeptoid hydration water structure and dynamics
Demonstrated that changes in both the number and placement of nonpolar monomers in the polypeptoid sequence affects both local water structure and dynamics and that such effects are correlated.
Quantifying polypeptoid conformational landscapes through integrated experiment and simulation
Proposed and cross-validated experimental and computational workflows to analyze structural populations of disordered polypeptoids.
Molecular Orientation and Structure in Model Ultrathin Layer-by-Layer Polyamide RO Membranes
Near Edge X-ray Absorption Fine Structure (NEXAFS) spectroscopy reveals molecular orientation varies with film thickness in layer-by-layer grown model RO membranes.
Simulations Reveal Complexity of Solute Affinity to Surfaces
Phase-field simulations demonstrate that mass-transfer-driven spinodal decomposition, thermal fluctuations, and structural arrest are essential to the formation of asymmetric polymer membranes in the Nonsolvent-Induced Phase Separation process.
Quantifying Polypeptoid Conformational Landscapes Through Integrated Experiment and Simulation
Proposed and cross-validated experimental and computational workflows to analyze structural populations of disordered polypeptoids.
End-to-end Distance Measurements of Aqueous Low-Molecular-Weight Polyethylene Oxide
Fully resolved end-to-end distance probability distribution measurements and simulations for aqueous polyethylene oxide (PEO) establish semi-flexible, excluded volume polymer.
Directly Probing Polymer Thin Film Chemistry and Counterions Influence on Water Sorption
Tender APXPS results provide direct quantification of hydration on polymers in situ at the molecular level.