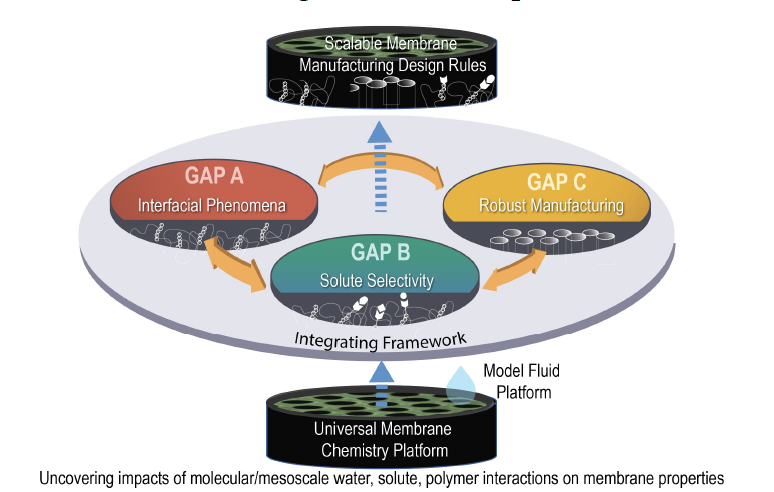
Integrating Framework (IF) for GAPS A, B, C: Bridging Between Systems/Developing the Bridging Toolset
IF Co-Leaders: Nate Lynd (UT), Phillip Christopher (UCSB)
IF Co-Investigators: Ethan Crumlin (LBNL), Craig Hawker (UCSB), Lynn Katz (UT), Dylan McReynolds (LBNL), Zak Page (UT), Rachel Segalman (UCSB), Todd Squires (UCSB), Greg Su (LBNL)
The Integrating Framework Provides the Foundation that Connects GAPs
M-WET interrogates fundamental processes underlying purification of fit-for-purpose water from alternative water sources via a highly interdisciplinary research coalition. The Integrating Framework (IF) will support and connect these wide-ranging research activities across M-WET by harmonizing the membrane materials, solutes, and characterization methods across the GAPs. The GAPs will create new molecular scale understanding of the structure and dynamics of water and solutes near membrane interfaces, design rules to control selectivity, and strategies for membrane formation. These fundamental discoveries will translate to fit-for-purpose polymer chemistries and significant improvements in macroscopic membrane performance. A key activity of the IF is the synthesis, scale-up, and documentation of the Universal Membrane Chemistry Platform (UMCP, Project 1). The IF will also support the GAPs by developing and utilizing characterization tools with in situ, in operando, (Projects 3 and 4) and highthroughput capabilities (Project 2) to guide synthesis, simulation, and theory. Finally, a membrane characterization team led by Lab Manager Kris Gleason will be responsible for more routine physical and chemical characterization of membrane properties including solute and water solubility, diffusivity, permeability, conductivity, dielectric properties, and mechanical properties of nonporous membranes. The team will also study flux, rejection, fouling, pore size, pore size distribution, surface energy, and mechanical properties of porous membranes. Vibrational spectroscopy, x-ray diffraction, and electron microscopy will also be employed to characterize virgin and fouled membranes. These bridging toolsets connect fundamental molecular-level membrane interactions and assemblies developed in the GAPs to system-level membrane separation performance and energy efficiency through tools that span length and time scales. Moreover, the multiscale suite of synchrotron X-ray tools are central to IF’s ability to translate and connect discoveries at the molecular level across GAPs and address separations across the MFP. The IF will enable M-WET to address Priority Research Directions relevant to multiple BES Basic Research Needs reports. It addresses PRDs from the Energy and Water BRN, including how to “achieve mechanistic control of interfaces and transport in complex and extreme environments.” Moreover, it addresses PRDs in the Transformative Manufacturing BRN, including (1) integrating multiscale models and tools to enable adaptive control of manufacturing processes and (2) unraveling the fundamentals of manufacturing processes through innovations in operando characterization. The IF’s goals are also in line with multiple PRDs outlined in the Transformative Experimental Tools BRN, such as (1) creating innovative experimental methods for investigating “real-world” systems and (2) simultaneously interrogating form and function, and bridging time, length, and energy scales.
The IF and M-WET team will develop and have access to unique characterization tools across length scales through multiple user facility proposals. This includes an ongoing Approved Program and general user proposals at the ALS, a general user proposal at the National Synchrotron Light Source II (NSLS-II), and a user project at the Molecular Foundry to directly simulate X-ray spectroscopy.
Project #1: Synthesis and Scale-up of UMCP Materials
The original porous UMCP platform has undergone an evolution into a more mechanically robust construct with a molecular architecture optimized for improved mechanical strength and non-equilibrium processing into isoporous morphologies. We use controlled anionic polymerization techniques to create a polystyrene-bpoly( ethylene-alt-propylene)-bpoly( styrene)-b-poly[(ethylene oxide)-co-(allyl glycidyl ether)] (PS1-b-PEP-b-PS2-b-P(EO-co- AGE)) material that exhibits substantially improved mechanical properties. We refer to the blocks sequentially as S1, EP, S2, and OX. Each component represents a distinct purpose with S1 providing a molecular anchor, EP inserting toughness and strength, S2 providing the requisite modulus to maintain a nanoscopic pore, and OX creating controlled hydrophilicity channels and functionality to attach interactants. Similarly within MWET we have developed a second non-porous UMCP platform based on copolymers of poly(ethylene glycol) acrylates and pentafluorophenyl acrylate (PFPA) that can be functionalized post-polymerization by a wide variety of functional groups (FG)40. The GAPs each address different portions of the UMCP architectures. GAP A focuses on understanding the role of FG on controlling water dynamics and solute interactions, GAP B focuses on the fundamentals of designing new membrane materials for selectivity by incorporating specific bonds, and GAP C focuses on the S1-EP-S2 subarchitecture.
Syntheses of new UMCP variants will be performed by a project scientist (TBD) supervised by Lynd and Page at UT using anionic and catalytic polymerization of vinyl and epoxide monomers for the porous platform and free radical routes for the non-porous platform. A 50% time project scientist at UCSB will focus on the functionalization of both porous and non-porous UMCP materials (supervised by Segalman and Hawker). A specialized array of reactor glassware will be made tailored to scale-up to a 100–1000 g scale. Materials will be synthesized, precipitated, dried, and placed in cold storage and will then be characterized within Project 3 for all parameters that affect processing and morphologyoverall molecular weight, molecular weight distribution, size of each block (S1, EP, S2, OX), and functional group (FG) identity. The molecular and morphological parameters will be stored in a database and made accessible to co-workers in all GAPs so that materials may be requested based on molecular, and also morphological characteristics, such as composition, molecular weight, pore size, spacing, morphology, and pore-size distribution as described in our data management plan.
Project #2: High Throughput Measurements of Membrane-solute Interactions
Making effective use of the UMCP material library to design functional membranes requires rapid screening of interactions with solutes. High throughput experiments will be used to characterize the sorption capacity, selectivity, and permeability of solutes at varying concentrations and feed complexity. Initial efforts to develop high throughput methodologies in M-WET have focused on: (a) UV-vis spectrophotometry on 96 well plates to measure solute sorption/desorption from hydrogels and (b) online robotic sampling approaches to measure membrane permeability of salt mixtures that have significantly different permeabilities and potential interactions between salt sorption processes.
As an example, we have synthesized ligand functionalized hydrogels based on the non-porous UMCP in a well plate format, with varying imidazole (ligand) concentrations. This array of hydrogels was then tested for sorption capacity of salts with varying concentrations (CuCl2, FeCl3, Cr(NO3)3 and K2CrO4). Spatially resolved UV-vis measurements of the hydrogel after exposure to the solute demonstrate the utility of high throughput analysis of the influence of hydrogel composition on the sorption capacity for various salts. The IF will develop the quantitative use of high throughput UV-vis spectroscopy to measure the thermodynamics and kinetics of the uptake of UV-vis active solutes onto libraries of materials based on the non-porous UMCP bearing ligands of interest (Christopher and Segalman).
M-WET has also developed an automated apparatus for measuring the permeability of mixed-salt feeds that enables rapid screening of solute transport through membranes via on-line robotic sampling. The apparatus also incorporates a remote, computer-controlled dosing of salt mixtures to a membrane for permeation studies. The system is designed to: (a) keep upstream salt concentrations fixed over time frames long enough to study salt mixtures where the components have widely varying permeability (e.g.., Li+ and Mg+2 mixtures) and (b) continuously replenish the receiver chamber to enable permeability measurements without influence of osmotic transport of water from the receiver to the donor chamber and allow time dependent variations in salt concentrations. From these data, ion permeability coefficients can be deduced. While the system was initially designed for automated, high throughput measurements of mixed salt permeabilities, it will also be used for automated measurements of the permeability of a single salt over a wide range of feed compositions, the permeability of mixtures with variations in concentrations of each salt, and conditioning analysis where hysteresis and long-time tests examine membrane fouling. Using a similar approach, we will develop an online ionic conductivity instrument, for example, using an electrochemical flow cell (Kristofer and Freeman).
Measurements made using these high throughput methodologies will guide membrane synthesis with targeted chemistries, facilitate detailed transport measurements by NMR (Clément), identify candidates for detailed analysis of fouling (IF Project #3), and provide benchmark experimental data for comparison to theoretical modelling (Ganesan).
Project #3: Operando Visualization of Membrane Fouling
One of the key areas of interest across the GAPs is identifying membrane functionality that promotes antifouling behavior as well as improving selectivity. Within the IF, Project 3 will work across GAPs to elucidate new fundamental understanding of membrane fouling with the model systems and UMCPderived membranes, providing critical chemical and nano- to mesoscale information to inform synthesis and theory efforts. The IF will determine the fouling resistance of novel membranes through fouling layer specific characterization and macroscopic membrane performance (Su, Crumlin, Katz, Kumar, Freeman).
M-WET’s groundwork studies to characterize membrane fouling reveal the importance of expanding to in operando and in situ characterization of fouling under realistic membrane operating conditions. Su and Katz will evolve their recently developed tender resonant X-ray scattering (TReXS) and resonant soft X-ray scattering (RSoXS) tools to characterize fouled RO membranes in situ during fouling. This will be coupled with element-specific near edge X-ray absorption fine structure (NEXAFS) spectroscopy to reveal molecular-level interactions in fouling layers. The IF will also develop pressurized crossflow cells that can be used for in operando scattering (SAXS/WAXS and SANS) and spectroscopy measurements (NEXAFS and EXAFS). Although previous work has used X-ray scattering to track mineral nucleation and growth and organic matter fouling, significant tangential flows and higher pressures are needed to mimic ultrafiltration and reverse osmosis conditions. Building off M-WET’s previous work, the well-defined form factors of cylindrical pores and spheres will allow scattering profiles to be fit to multiple population models to distinguish between internal and external fouling and independently track the contribution of each during the fouling process. Real-time tracking of fouling layer buildup will provide information on how speciation and nanoscale distributions evolve with exposure to complex waters across the MFP, focusing particularly on neutral and ionic foulants and surface functionalizations being studied in GAP A. Real-time fouling studies will begin with hard Xray scattering and spectroscopy measurements, which are performed in ambient conditions. In situ resonant scattering (RSoXS and TReXS) will be pursued simultaneously and will leverage recent developments in vacuum-compatible flow cells. This will provide new experimental capabilities to track membrane fouling and fouling layer nanostructure with chemical sensitivity. Operando nanoscale characterization using crossflow cells that mimic macroscopic membrane measurements will provide the bridge between membrane chemistry and performance.
The IF’s operando measurements will be guided by theory and simulation. M-WET has leveraged direct simulations of NEXAFS spectroscopy using the Many-Body X-ray Absorption Spectroscopy (MBXAS) methodology based on density functional theory to understand local environments around specific foulingrelevant species, e.g., calcium-carboxyl interactions. The IF will continue to advance quantum chemical calculations and combine this with atomistic and coarse-grained simulations based on expertise in GAPs B and C (Fredrickson, Henkelman, Ganesan) to correlate measured spectroscopic and scattering data to molecular-scale microenvironments and nanoscale phases.
Project #4: Operando Characterization of Assembly Pathways in Integral Asymmetric Membranes
While GAP C will utilize the UMCP block polymer library to understand and develop the formation process of isoporous asymmetric membranes via SNIPS/NIPS, in the IF, Crumlin, Katz, and Su will develop the unique and critical operando scattering tools necessary to track each stage of the SNIPS process. As discussed in GAP C, the process by which block copolymers are processed to form isoporous asymmetric membranes is a non-equilibrium process that is empirically optimized for each new chemistry. Via the development of operando sample stages and high sensitivity/speed detectors capable of capturing micro to millisecond snapshots for neutron and hard X-ray scattering, the IF will develop tools that can uniquely monitor both the initial solvent casting of the block copolymer micelles and then the non-solvent immersion processes that comprise SNIPS and whose mechanism has heretofore been the subject of intense speculation. The high flux of synchrotron X-rays will provide fast snapshots during the critical 15–60 seconds after solvent evaporation begins during the membrane casting process. The desired 10–100 nm characteristic length scale in the isoporous skin layer is ideal for the length scales SAXS can probe. Moreover, in situ grazing incidence SAXS (GISAXS) will enable enhanced surface sensitivity, which is relevant to the isoporous layer that exists only in the top few hundred nanometers of the membrane. Further, we will leverage and advance recent developments at the ALS in in situ liquid resonant soft X-ray scattering (RSoXS) to develop ways to probe polymer micelles and precisely discriminate the spatial extent of the core vs. shell regions of micelles based on the ability to selectively enhance contrast between polymer blocks by tuning the incident X-ray energy. A second sample cell will be developed to follow the immersion process through which an open porous structure is developed during SNIPS/NIPS. Moreover, by combining GISAXS with transmission SAXS, structure formation of features of up to 100s of nanometers can be tracked on the membrane surface and through the membrane thickness.
Integral asymmetric isoporous membranes can also be fabricated in a hollow fiber geometry. This geometry is significant since the hollow fiber is one of two (along with flat sheet) dominant form factors used for current membranes. In situ SAXS with the X-ray beam positioned at various distances from the spinning nozzle will provide information on the kinetics of structural rearrangements after extrusion. M-WET will expand past structure formation of flat sheet membranes to hollow fiber geometries and leverage our access to multiple SAXS beamlines at the ALS and NSLS-II with varying casting-conditions of pre-formed membranes to connect processing conditions to membrane characteristics. The novel UMCP platform will provide a route towards isoporous membranes with functionalizable hydrophilic pore walls. Synchrotron infrared nanospectroscopy (SINS) and resonant X-ray scattering will be used to map nanoscale morphology with chemical sensitivity, through unique IR signatures of specific functional groups and tunable scattering contrast, respectively. This will also provide a route to spatially resolve the distribution of adsorbed solutes in membranes exposed to complex waters. Thin films cast from micellar solutions have been shown to attain morphologies similar to thicker membranes, and this will be ideal for transmission RSoXS studies to decipher the distribution of each domain in a phase separated multiblock copolymer and the impacts of hydration.
Development of next-generation membranes requires co-design of selectivity, permeability, and mechanical properties (Transformative Manufacturing BRN PRD 4: Co-design materials, processes, and products to revolutionize manufacturing). Despite great advances in new polymer chemistries to improve transport and selectivity, concurrent optimization of mechanical properties remains an open research question. Previous work has shown that for a pentablock copolymer with an ion-containing sulfonated midblock, the creep behavior depends strongly on the degree of sulfonation, molecular weight of the sulfonated block, and end-block chemistry. However, the impact of membrane morphology, e.g., discrete vs. interconnected ionic domains, on mechanical properties remains largely unknown. GAP C (Sanoja) will provide foundational knowledge of polymer mechanical properties and merge with the operando characterization developments in IF to simultaneously track membrane mechanics and morphology. The ALS has tensile testing stages for the SAXS/WAXS beamline and a vacuum-compatible tensile tester for the RSoXS beamline. These will serve as a starting point for in situ scattering measurements during stretching, which will provide new insights into how morphological parameters, e.g., domain size and separation, influence mechanical behavior. M-WET will further develop custom sample cells specific to isoporous membranes.
Integrating Framework
-
Phillip Christopher
IF Co-Leader
-
Ethan Crumlin
GAP A Co-Investigator, IF Co-Investigator
-
Craig Hawker
IF Co-Investigator
-
Lynn Katz
Associate Director, GAP A Co-Leader, GAP B Co-Investigator, GAP C Co-Investigator, IF Co-Investigator
-
Nate Lynd
GAP A Co-Investigator, GAP C Co-Investigator, IF Co-Leader
-
Dylan McReynolds
IF Co-Investigator
-
Zak Page
IF Co-Investigator
-
Rachel Segalman
Associate Director, GAP A Co-Investigator, GAP B Co-Leader, IF Co-Investigator
-
Todd Squires
IF Co-Investigator
-
Greg Su
GAP A Co-Investigator, GAP C Co-Investigator, IF Co-Investigator
Research Highlights - IF
A unified two-phase membrane transport model
Reconciles two classical model descriptions of membrane transport for swollen non-porous membrane using the Fluid-solid model, a unified mechanical framework to approach solvent transport.
Salt and ion transport in a series of crosslinked AMPS/PEGDA hydrogel membranes
Fundamental ion transport properties in membranes synthesized with either the same charge density or the same water uptake were characterized. These properties were compared to established models to build structure-property relationships.
Designing Solute-Tailored Selectivity in Membranes
New synthetic strategies to improve membrane selectivity were identified and fundamental design questions were addressed.
Electrodialysis Pretreatment to Improve Boron Removal
Selective removal of divalent cations (e.g., Ca+2) via electrodialysis prior to nanofiltration reduces membrane fouling, which reduces permeate flux decline and improves boron rejection.
Separation Characteristics of Cations and Natural Organic Matter in Electrodialysis
Identifies a hybrid electrodialysis (ED)-Nanofiltration (NF)/reverse osmosis (RO) system and identifies appropriate properties of ion exchange membranes of ED pretreatment for water sources that require little or no desalination but have high NOM concentrations.